Phiala Shanahan is seeking fundamental answers about our physical world
With supercomputers and machine learning, the physicist aims to illuminate the structure of everyday particles and uncover signs of dark matter
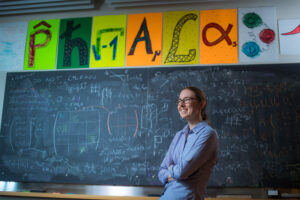
Associate Professor Phiala Shanahan’s research focuses on particle and nuclear theory and applying machine learning to understand the fundamental interactions of nature. Photo: Gretchen Ertl
In 2010, Phiala Shanahan was an undergraduate at the University of Adelaide, wrapping up a degree in computational physics, when she heard of an unexpected discovery in particle physics. The news had nothing to do with any of the rare, exotic particles that physicists were searching for at the time. Rather, the revelation revolved around the mundane, ubiquitous proton.
That year, scientists had measured the proton’s radius and discovered that the particle was ever so slightly smaller than what previous experiments had reported. This new measurement threw into question what physicists had assumed was well-understood: What exactly was the size of the proton?
What would then be coined the “proton radius puzzle” immediately drew Shanahan’s interest, prompting a more fundamental question: What else don’t we know about this seemingly straightforward particle?
“Protons and neutrons make up 99 percent of visible matter in the universe,” she says. “I assumed that, just like the mass of the proton is known very precisely, the size would be too. That was one moment fairly early on when I realized, there really are fundamental questions that we still have no answers to.”
The proton puzzle was one impetus that propelled Shanahan to pursue theoretical particle and nuclear physics. Today, she is the Class of 1957 Career Development Associate Professor of Physics at MIT, having recently received tenure at the Institute.
In her research, she seeks a fundamental understanding of our physical world. Using the equations of the Standard Model of Physics as her guide, she is looking for fundamental bridges — concrete, mathematical connections between the behavior of elementary particles, such as the quarks and gluons within a single proton, and the interactions between multiple protons, which coalesce into the visible matter we see around us.
Tracing these fundamental connections will ultimately help physicists recognize breaks in our understanding, such as instances when a proton interacts with dark matter, which is thought to make up 85 percent of the total mass in the universe and for which the Standard Model — our best representation of our physical understanding — has no explanation.
“We are trying to understand how you can bridge understanding from our most fundamental theory — this beautiful predictive theory of fundamental particles — all the way up to nuclear physics,” Shanahan says.
Up for a challenge
Shanahan was born in Sydney, Australia, and spent most of her childhood and early education in the suburbs of Adelaide, where she earned a scholarship to attend an all-girls school. She quickly took to studying math and science, learning new languages, and playing a variety of instruments.
“At the time, I don’t think you could’ve picked me for a scientist rather than a musician or a linguist,” she says.
After high school, Shanahan stayed local, attending the University of Adelaide, where she took classes in Latin and ancient Greek, and played in a cover band on the weekends. She also pursued a bachelor’s degree in high-performance computational physics, which she chose almost as a personal challenge.
“It was the hardest degree to get into at the time, and I thought, ‘I want something challenging,’” she recalls.
Her interest in physics began to crystallize after hearing of the proton radius puzzle one day in a research seminar. She also discovered that she enjoyed research, after accepting an offer to work as a summer assistant in the lab of her undergraduate advisor, Anthony Thomas, who specialized in nuclear physics. She continued working with Thomas through graduate school, also at the University of Adelaide, where she earned a PhD in theoretical nuclear physics.
“I’d already been caught by this idea that we didn’t know nearly as much about the proton as I thought, so my PhD was about understanding in great detail the structure of the proton and what we could add to that understanding,” Shanahan says.
A direct trace
After finishing her education in Australia, Shanahan looked to take her next step, outside the country. With funds from a traveling fellowship, she planned out a two-month tour of physics departments and facilities across Europe and the United States, including at MIT. The experience was a whirlwind, as Shanahan was introduced at every stop to new ideas and avenues of research.
“The mind expansion was really exciting,” she says.
When she came home to Australia, she found she was keen to keep on the research track, and to live abroad. Soon, she packed her bags for a postdoc position in MIT’s Department of Physics. She arrived at the Institute in 2015 and spent the next two years researching the interactions of gluons, the elementary, force-carrying particles that bind to quarks to form a proton.
“It’s very difficult to measure experimentally certain aspects of the gluon structure of a proton,” Shanahan says. “I wanted to see what we could calculate, which at the time was quite a new thing.”
Until then, Shanahan considered herself a mostly “pen- and-paper” theorist. But she wanted to see how far the behavior of gluons — interactions known as quantum chromodynamics — could be directly traced using the equations of the Standard Model. To do so would require large-scale numerical calculations, and she found herself learning a new set of computational tools and exploring ways to search for fundamental interactions among gluons using machine learning — a novel approach that Shanahan was one of the first to adopt, and which she continues to pursue today.
A creative space
After finishing her postdoc, she spent a year as a faculty member at the College of William and Mary and as a senior staff scientist at the Thomas Jefferson National Accelerator Facility before returning to MIT in 2018 as an assistant professor in the Center for Theoretical Physics. Before she put down campus roots, Shanahan spent the fall semester at the Perimeter Institute for Theoretical Physics in Ontario, Canada, as part of a fellowship that supports female physicists. The program provided food and board for fellows, and also delivered meals to their offices — all with the goal of freeing the physicists to focus on their work.
“That program really gave me the launchpad for what became my research agenda as a new faculty member,” she says. “It all started from that quiet time where I could actually think for hours at a time. That was incredibly valuable, and it gave me the space to be creative.”
At MIT, she continues to study the equations of the Standard Model to understand the quantum dynamics of gluons and quarks, and the structure of the proton, as well as the interactions that underpin nuclear physics, and what the fundamental behavior of certain nuclei can tell us about the conditions of the early universe.
She is also focusing on nuclei that are used in dark matter experiments, and is looking to map out the space of nuclear interactions that can be explained concretely through the Standard Model. Any interactions outside of this fundamentally derived space could then be a sign of dark matter or other phenomena beyond what the Standard Model can explain.
“Now my research group is going in all sorts of directions,” she says. “We are using every tool at our disposal, from pen-and-paper calculations, to designing and running new algorithms on supercomputers, to really understand new aspects of the structure and interactions of the matter that makes up our universe.”
Jennifer Chu | MIT News Office
This article first appeared in MIT News on February 28, 2023.