If you build it…
The MIT Kavli Institute (MKI), led by Director Professor Robert Simcoe, equips people and laboratories to build customized experiments to study the universe. MKI’s astronomical instrumentation experts work in three major areas: discovering exoplanets, characterizing the first stars and galaxies, and understanding environments with extreme gravity, such as black holes and neutron stars. In this issue of Science@MIT, we explore instruments led by MKI researchers that you might not yet know about.
It’s outta here
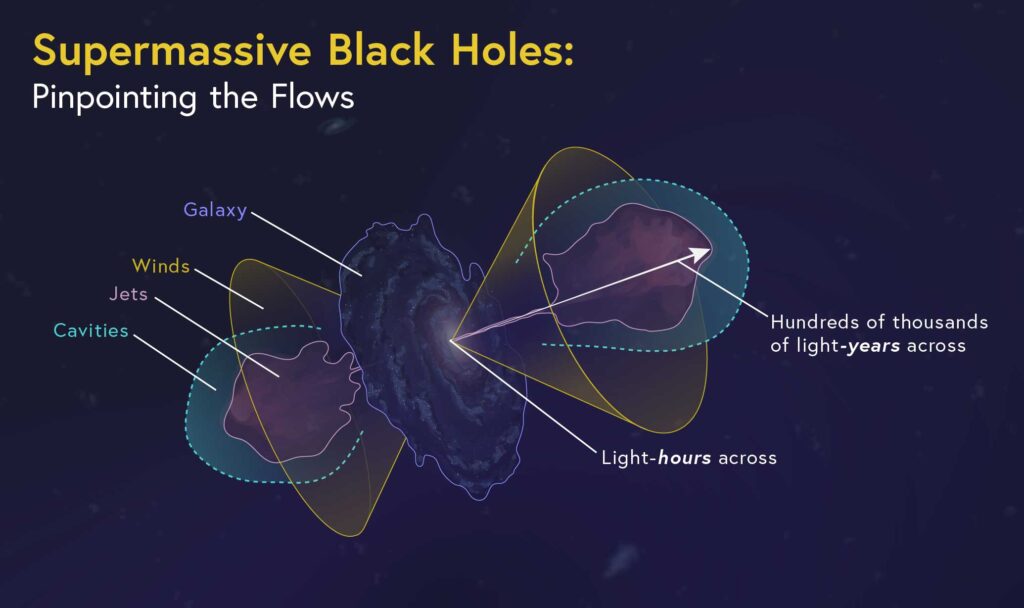
While most — and perhaps all — galaxies have supermassive black holes at their centers, active galaxies are unique in that their supermassive black holes are actively gobbling up gas and dust from their surroundings. This process releases enormous amounts of energy and results in some of the most energetic phenomena in the universe. Many of these active galaxies eject high-temperature plasma, or jets, at nearly the speed of light, producing X-rays in their magnetic fields. X-rays, like all forms of light, can be polarized. And just like polarized sunglasses can reduce glare on a sunny day, an X-ray polarimeter instrument can detect the glare of X-rays produced by plasma.
NASA recently approved a sounding rocket mission that will fly an MIT-built X-ray polarimeter to study a single active galaxy during the rocket’s five minutes above the atmosphere. The mission’s lead investigator, Herman L. Marshall, a MKI principal research scientist, has designed the astrophysics instrument to measure the polarization of the X-rays from these active galaxies in order to understand the structure and formation of these active galaxy jets.
Dubbed the Rocket Experiment Demonstration of a Soft X-ray Polarimeter, the REDSoX Polarimeter uses advanced multilayer coated mirrors and highefficiency diffraction gratings — technology also pioneered at MIT — to study the polarization of low-energy, or “soft,” X-rays from active galaxies. The technique demonstrated by the REDSoX team, that includes MKI research scientist Sarah Heine and postdoc Alan Garner, will pave the way for future orbital missions using this MIT technology to study these fascinating objects.
CHIME
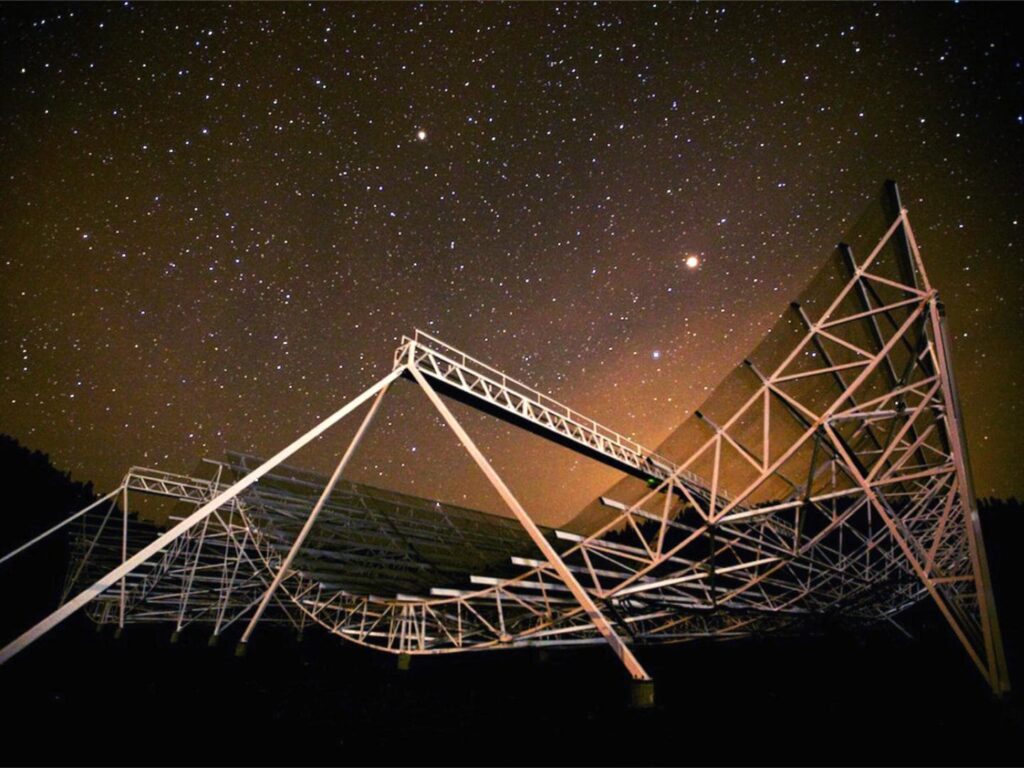
The Canadian Hydrogen Intensity Mapping Experiment, or CHIME, is an interferometric radio telescope located at the Dominion Radio Astrophysical Observatory in British Columbia, Canada. Though CHIME was designed to pick up radio waves emitted by hydrogen, the telescope is also sensitive to fast radio bursts.
Fast radio bursts (FRBs) are bright, brief flashes of light, registering in the radio band of the electromagnetic spectrum. The origins of these millisecond bursts are unknown, and their appearance is unpredictable. Since the first FRB was discovered in 2007, astronomers had only recorded around 140 bursts. Since CHIME began observing in 2018, the instrument has detected hundreds of FRBs emanating from different parts of the sky.
As a radio wave travels across space, any interstellar gas, or plasma, along the way can distort or disperse the wave’s properties and trajectory. The degree to which a radio wave is dispersed tells how much gas it passed through, and can give clues to how far it has traveled from its source.
Most recently, Assistant Professor Kiyoshi Masui and others on the CHIME team, discovered the longest-lasting FRB, which was made up of a series regularly spaced bursts. The team suspects the signal might be from a rapidly spinning object, such as a pulsar or a magnetar. At MKI, Masui is building the correlator for an expanded network of radio telescopes across North America. This new network will pinpoint the exact sky locations where FRBs are triggered.
Ping me: CHIME has a large instantaneous field of view (200 square degrees) and broad frequency coverage (400-800 MHz). This is enabled by its digitally-driven design: it has no moving parts and points to many sky locations simultaneously using digital signal processing and a large computer cluster. These capabilities allow scientists to make a 3D map of hydrogen density — and, as a bonus, can provide information on fast, transient radio emissions.
Fourth wave technology
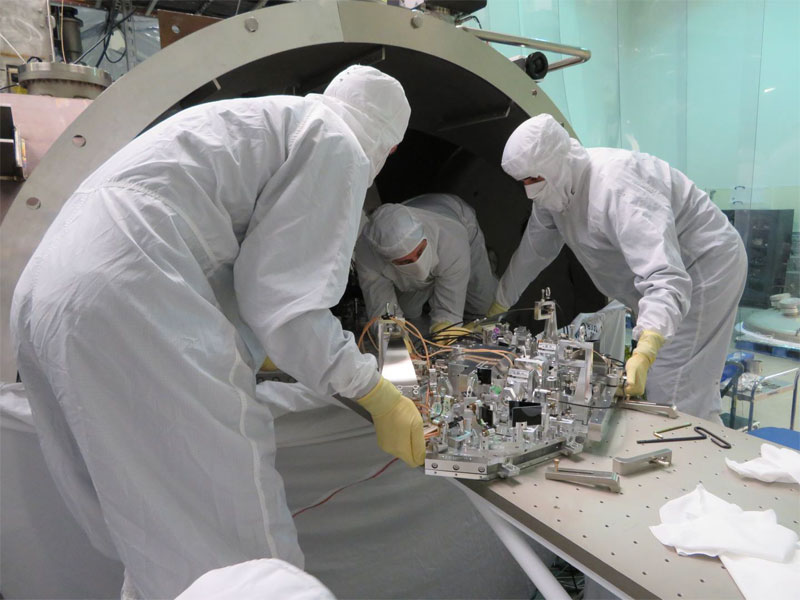
In 2015, the Advanced Laser Interferometer Gravitational-Wave Observatory (LIGO) made the first detection of gravitational waves from a binary black hole system. By the end of the second observing run (O2) in August 2017, the LIGO instruments and the Virgo interferometer in Italy recorded 11 gravitational-wave signals, including one from a binary neutron star merger. The third observing run (O3) started in April 2019, with improved LIGO and Virgo detectors and the addition of the Japanese KAGRA observatory in 2020.
Though O3 ended abruptly with the start of the pandemic, the MIT-designed instrumentation called a “quantum squeezer” was already tamping down the quantum noise generated by the vacuum fluctuations (zero-point energy) that enter the LIGO detectors. The reduced quantum noise in the interferometers contributed to increase the detection rate of gravitational-wave signals from approximately once per month in O2 to once per week in O3.
The last two years have seen major upgrades to LIGO and Virgo instruments, including the addition of a 300-meter-long filter cavity that optimally manipulates squeezed light before injection in the interferometers. These upgrades will help to make the most sensitive detectors yet, with a projected two-to three-fold increase in detection rate in the upcoming fourth observing run (O4) with respect to O3.
MKI Senior Research Scientist Lisa Barsotti has led the development of the LIGO quantum squeezer for over a decade. With the resulting upgraded technologies, coupled to other detector improvements, the LIGO Scientific Collaboration projects detecting binary star mergers at a distance of 160 to 190 megaparsecs in O4 (scheduled to start in March 2023, and last for one year), more than twice the distance reported in O1.
Cosmic Explorer
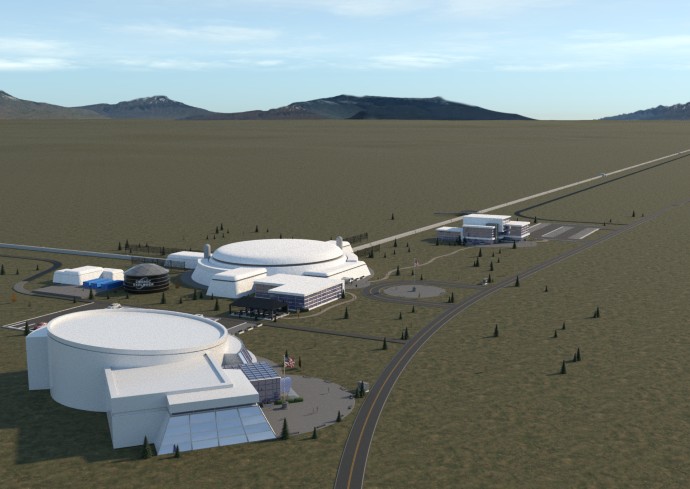
MathWorks Professor of Physics Matthew Evans is leading the U.S. efforts to develop Cosmic Explorer, a proposed next-generation gravitational-wave observatory, that will allow a full order of magnitude sensitivity improvement beyond Advanced LIGO.
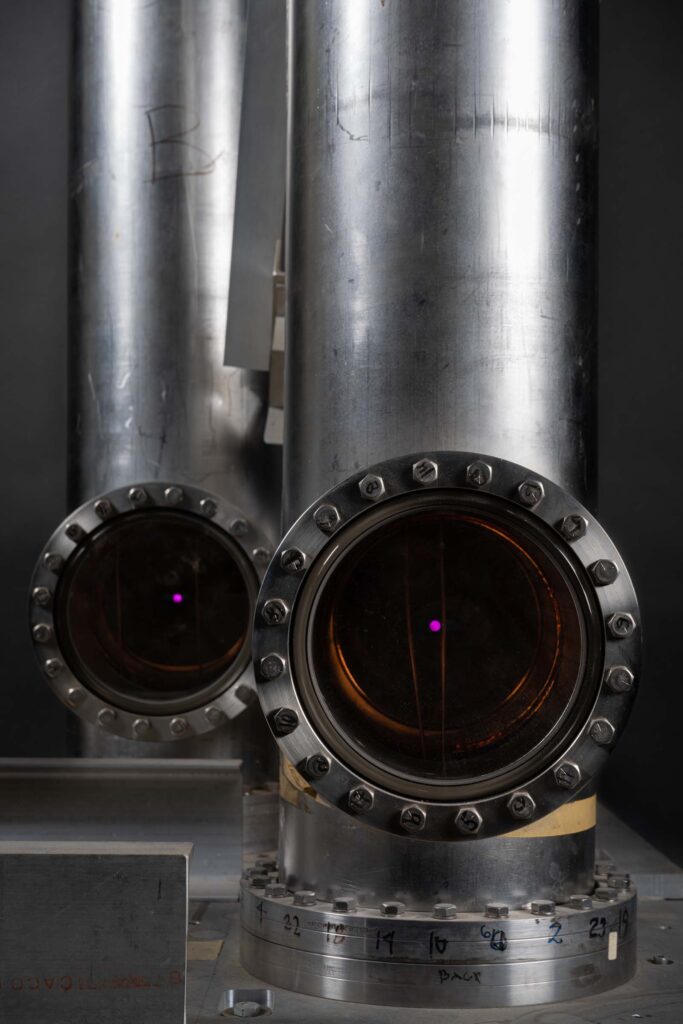
Like the current generation of observatories, Cosmic Explorer observatories will have an L-shaped geometry and a single interferometer. Although there are areas of detector technology where improvements will lead to increases in the bandwidth and sensitivity (such as a higher-performance quantum squeezer), the dominant improvement comes from the increased arm length; they will be five to 10 times that of the Advanced LIGO facilities!
This means Cosmic Explorer will leap from only monitoring the nearby universe to surveying the entire universe for black hole and neutron star mergers. This increase will allow the observatories to peer further back in history and gravitational-wave signals from epochs when star formation was still in its infancy.
In addition to improved high-fidelity signals and greater redshift reach, Cosmic Explorer and next-generations of ground-based gravitational-wave observatories may allow researchers to detect dark or exotic matter around black holes, to test modified theories of gravity, or test astrophysical scenarios of compact-object formation and evolution.
LLAMAS
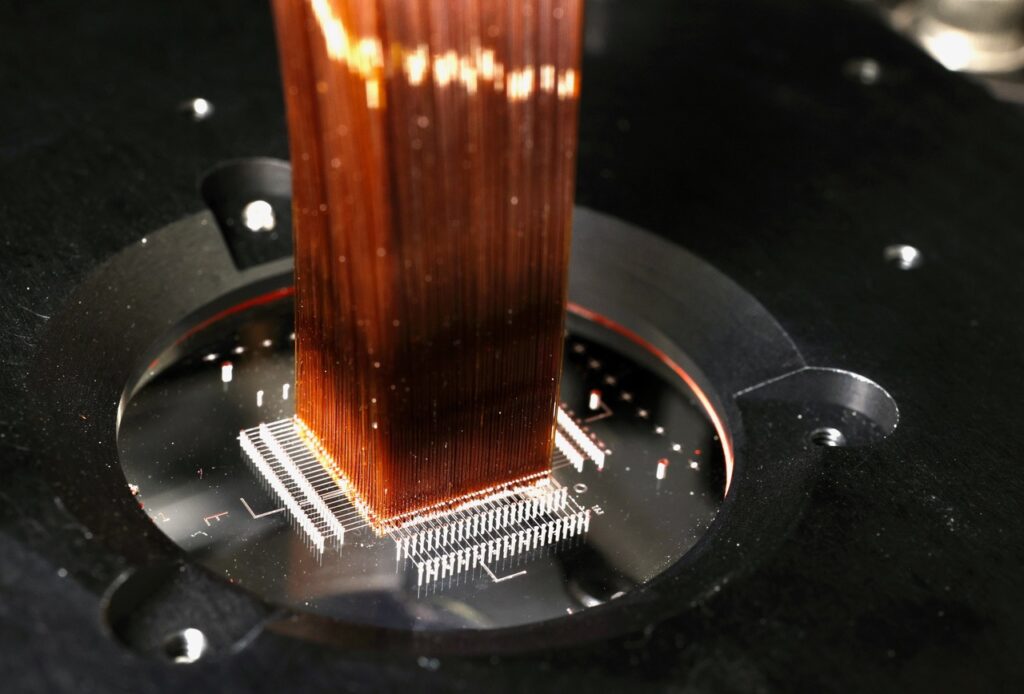
The Large Lenslet Array Magellan Spectrograph (LLAMAS) is a new 3D imager scheduled for 2023 installation at the 6.5-meter Magellan Telescopes at the Las Campanas Observatory in Chile. The design and construction of LLAMAS is being led by Professor Robert Simcoe and MKI principal research scientist Gabor Furesz.
LLAMAS injects starlight from the telescope into a fine grid of optical fibers, recording optical spectra with 6,000 colors at each of 2,400 discrete sky positions. These are used to reconstruct a 3D image of the sky with photon wavelength as the depth dimension.
LLAMAS utilizes two primary subsystems: an bank of eight spectrographs that disperse and record the color traces for each fiber, and a micro-lens array that couples light between the telescope and fibers. To facilitate this technically challenging endeavor, the team cut and packaged more than 3,000 fibers at home during the Covid shutdown, and built a custom tool to align and bond each fiber to its correct position in the telescope focus, within a fine tolerance of a few microns.
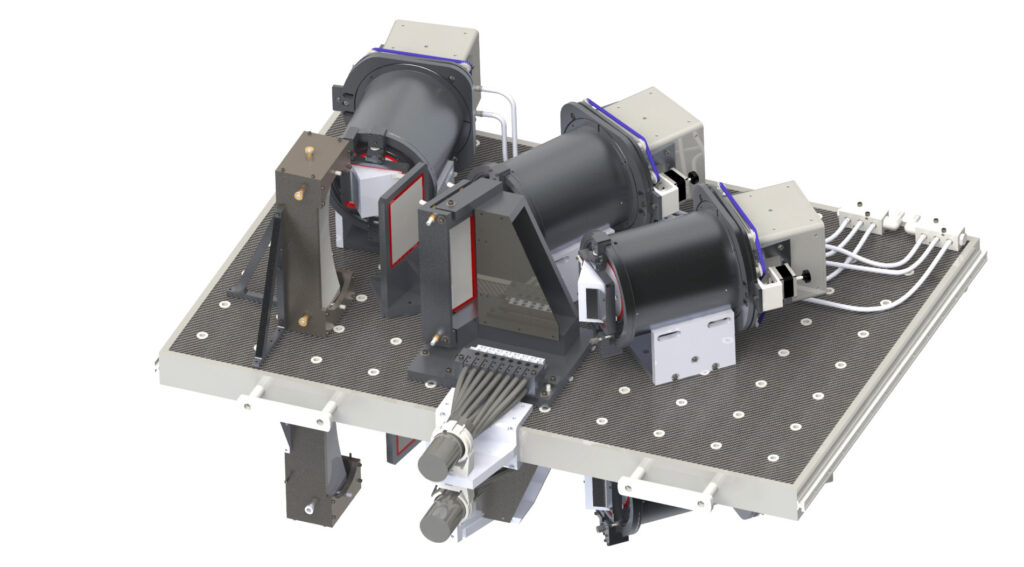
Many key survey telescopes are being built in the Southern Hemisphere, including the Vera Rubin Observatory, the South-Pole Telescope, and the Atacama Large Millimeter/submillimeter Array. LLAMAS will work in concert with these observatories, providing MIT and other U.S. astronomers with wideband 3D views of the tens of thousands of sources these facilities will uncover.